GRIGNARD REAGENT
Reaction Mechanism of Grignard Reagent
The Grignard reagent, we recall, has the formula RMgX, When a solution of an alkyl halide in dry ethyl ether, (C2H5)2O, is allowed to stand over turnings of metallic magnesium, a vigorous reaction takes place. The solution turns cloudy, begins to boil and the magnesium metal gradually disappears. The resulting solution is known as a Grignard reagent. It is one of the most useful and versatile reagents known to the organic chemists… This halide can be alkyl (1o, 2o, 3o), allylic, aryl alkyl (e.g. benzyl), aryl (phenyl) or substituted phenyl. The halogen may be –Cl, –Br or –I, (Arylmagnesium chlorides must be made in the cyclic ether tetrahydrofuran instead of ethyl ether.)
Aldehydes and ketones resemble each other closely in most of their reactions. Like the carbon-carbon double bond, the carbonyl group is unsaturated, and like the carbon-carbon bond, it undergoes addition. One of its typical reactions is addition of the Grignard reagent.
The carbon-magnesium bond of the Grignard reagent is a highly polar bond, carbon being negative relative to electropositive magnesium. It is not surprising, then, that in the addition to carbonyl compounds, and the organic group becomes attached to carbon and magnesium to oxygen. The product is the magnesium salt of the weakly acidic alcohol and is easily converted into the alcohol itself by the addition of the stronger acid, water. Since the Mg(OH)X thus formed is a gelatinous material difficult to handle, dilute mineral acid (HCl, H2SO4) is commonly used instead of water, so that water-soluble magnesium salts are formed.
The action of Grignard’s reagent on aldehydes, ketones and esters
Grignard Reagent use
The Grignard reagent is the best-known member of a broad class of substances called organometallic compounds, in which carbon is bonded to a metal like lithium, potassium, sodium, zinc, mercury, lead, thallium or to almost any metal known. Each kind of organometallic compound has, of course, its own set of properties and its particular uses depend on these. But whatever the metal, it is less elctronegative than carbon, and the carbon-metal bond-like one in the Grignard reagent - is highly polar. Although the organic group is not a full-fledged carbanion–an anion in which carbon carries negative charge, nevertheless has considerable carbanionic character. As we shall see, organometallic compounds owe their enormous usefulness chiefly to one common quality, they can serve as a source from which carbon is readily transferred with its electrons.
The Grignard reagent, we recall, has the formula RMgX, and is prepared by the reaction of metallic magnesium with the appropriate organic halide. This halide can be alkyl (1°, 2°, 3°), allylic, aryl alkyl (e.g. benzyl), or aryl (phenyl) or substituted phenyl. The halogen may be –Cl, –Br or –I, (Arylmagnesium chlorides must be made in the cyclic ether tetrahydrofuran instead of ethyl ether).
Aldehydes and ketones resemble each other closely in most of their reactions. Like the carbon-carbon double bond, the carbonyl group is unsaturated and like the carbon-carbon bond, it undergoes addition. One of its typical reactions is addition of the Grignard reagent.
Since the electrons of the carbonyl double bond hold together atoms of quite different electronegativity, we would not expect the electrons to be equally shared, in particular, the mobile π cloud should be pulled strongly towards the more electronegative atom, oxygen. Whatever the mechanism involved, addition of an unsymmetrical reagent is oriented so that the nucleophilic (basic) portion attaches itself to carbon and the electrophilic (acidic) portion attaches itself to oxygen.
The carbon-magnesium bond of the Grignard reagent is a highly polar bond, carbon being negative relative to electropositive magnesium. It is not surprising, that in the addition to carbonyl compounds, the organic group attached to carbon and magnesium to oxygen.
The product is the magnesium salt of the weakly acidic alcohol and is easily converted into the alcohol itself by the addition of the stronger acid, water. Since the Mg(OH)X thus formed is a gelatinous material difficult to handle, dilute mineral acid (HCl, H2SO4) is commonly used instead of water, so that water-soluble magnesium salts are formed. Exceptional behavior of Grignard Reagent In certain cases, a Grignard reagent does not react with compounds containing functional group normally capable of reaction. Generally the branching of the carbon chain near the functional group prevents the reaction, the cause is probably the steric effect. For example, methyl magnesium bromide or iodide does not react with hexamethyl acetone (CH3)3CCOC(CH3)3. It has also been found that if Grignard reagent contains large alkyl groups, reaction may be prevented. For example, isopropyl methyl acetone reacts with methyl magnesium iodide but not with t-butyl magnesium chloride. In other cases, abnormal reaction may take place. For example, when isopropyl magnesium bromide is added to diisopropyl ketone, the expected tertiary alcohol is not formed, instead the secondary alcohol, di-isopropylcarbinol is obtained resulting from the reduction of the ketone.
(CH3)2CHCOCH(CH3)2 (CH3)2CHCH(OH)CH(CH3)2 + CH3CH = CH2
α, β unsaturated carbonyl compounds react with Grignard reagent in the 1,2-or 1,4-positions.
1,2 addition In Grignard Reagent
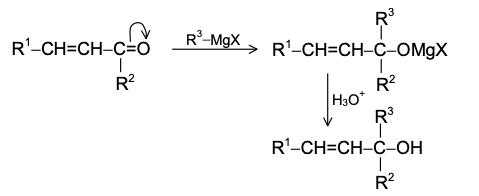
1,4 addition:
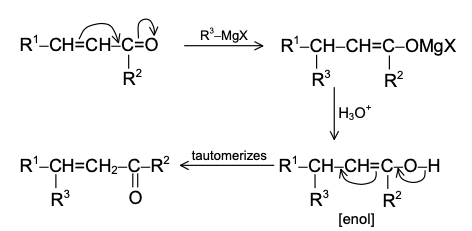
The extent of 1,4 addition can be made greater by the use of Cu2Cl2 or (CH3COO)2Cu. In this case, reaction is initiated by the free radical mechanism.The reaction of dihalides of the type Br(CH2)nBr with magnesium depends on the value of n. For n = 1-3, no Grignard reagent is formed. For example,

When n ≥ 4, the Grignard reagent can be possibly made with dibromo compound viz, Br(CH2)nBr.
Products of the Grignard Synthesis
The class of alcohol that is obtained from a Grignard synthesis depends upon the type of carbonyl compound used. Formaldehyde (HCHO) yields primary alcohols, other aldehydes (RCHO) yield secondary alcohols and ketones (R2CO) yield tertiary alcohols.This relationship arises directly from our definitions of aldehydes and ketones and our definitions of primary, secondary and tertiary alcohols. The number of hydrogens attached to the carbonyl carbon defines the carbonyl compound as formaldehyde, higher aldehyde or ketone. The carbonyl carbon is the one that finally bears the –OH group in the product and the number of hydrogen defines the alcohol as primary, secondary, or tertiary.
For example,
Planning a Grignard Synthesis
How do we decide which Grignard reagent and which carbonyl compound to use in preparing a particular alcohol ? We have only to look at the structure of the alcohol we want. Of the groups attached to the carbon bearing the –OH group, one must come from the Grignard reagent, the other two (including any hydrogens) must come from the carbonyl compound.
Most alcohols can be obtained from more than one combination of reagents. We usually choose the combination that is most readily available. For example, consider the synthesis of
2-phenyl-2-hexanol.
As shown, we could make this either from the four-carbon Grignard reagent and the aromatic ketone, or from the phenyl Grignard reagent and the six-carbon aliphatic ketone. As we know, the first route uses the more readily available carbonyl compound and is the one actually used to make this alcohol.
Grignard reagent from a compound (e.g., HOCH2CH2Br) that contains, in addition to halogen, some group (e.g.,–OH) that will react with a Grignard reagent. If the preparation of Grignard from this were tried, as fast as a molecule of Grignard reagent formed, it would react with the active group (–OH) in another molecule to yield an undesired product (HOCH2CH3).
We must be particularly watchful in the preparation of an arylmagnesium halide, in view of the wide variety of substituents that might be present on the benzene ring. Carboxyl (-COOH), hydroxyl (-;OH), amino (-NH2) and -SO3H all contain hydrogen atom attached to oxygen or nitrogen and therefore are so acidic that they will decompose a Grignard reagent.
The nitro (-NO2) group oxidizes a Grignard reagent. It turns out that only a comparatively few groups may be present in the halide molecule from which we prepare a Grignard reagent, among these are -R, -Ar, -OR and -Cl (of an aryl chloride).The aldehyde (or other compound) with which a Grignard reagent is to react may not contain other groups that are reactive towards a Grignard reagent. For example, a Grignard reagent would be decomposed before it could add to the carbonyl group of following compounds
A related synthesis utilizes ethylene oxide to make primary alcohols containing two more carbons than the Grignard reagent.
Here, too, the organic group attaches to carbon and magnesium to oxygen. In this reaction breaking of carbon-oxygen σ bond takes place in the highly strained three-membered ring. For example,’